Contatto Editoriale:
Paolo Lista,
Lista Studio srl®
Borgo Belvigo 33, 36016 Thiene Vi ITALY
tel/fax 0445,372479 o info@lista.it
Vehicle - cyclist crash analysis
Prof. Gabriele Virzì Mariotti and eng. Filippo Carollo (University of Palermo, Italy), together with dr. Edoardo Scalici (Dipartimento di Biopatologia e Biotecnologie Mediche e Forensi), have presented in November 2014 in Florence, Italy, at the 14th ECME a paper on Biomechanics Parameters in the Vehicle-Cyclist Crash with Accident Analysis in Palermo, here summarized with permission from the Authors.
Summary
The vehicle-bicyclist impact is studied in this paper. After analysis of the accidents in Palermo in the last years, the multibody simulation of the crash is executed by making use of SimWise 4D (alias visualNastran 4D simulation software). Dummy, car and bicycle are those used in previous works presented in this showcase, as in the vehicle-pedestrian crash analysis, even in the case of a teenager, with relevant anthropomorphic model. The attention is on a teenage cyclist, because the data on this argument are found in literature with difficulty. The impact is simulated at four different speeds until 50 km/h, with three different positions of the cyclist relative to the vehicle: frontal, side and rear. In particular the injury of the head is analyzed using the parameter HIC and the chest injury is analyzed by 3 ms criterion; the likelihood AIS 4+ is calculated, concluding that head injury is more dangerous in the case of teenage pedestrian, while chest injury is more dangerous in the case of the cyclist.
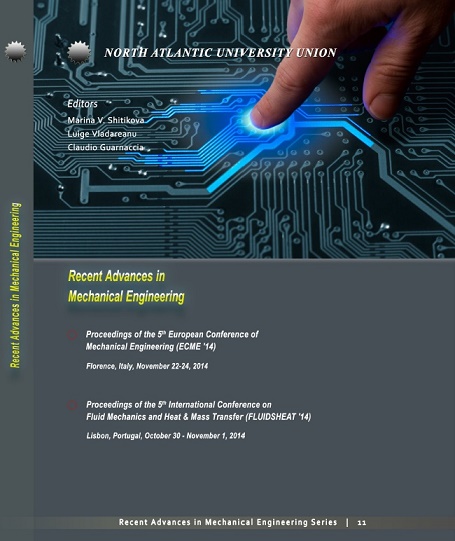
1. Introduction
Every day in Italy a cyclist loses the life, and other cyclists show more or less serious wounds, requiring hospitalization. The numbers show a true emergency because there are at least 1,000 deaths in the last 3 years. The risk of mortality, by calculating the average value of 1, for cyclists is 2.18, more than double of the base value. Mortality rate is equal to 0.78 for the cars, 0.67 for the trucks,0.48 for buses, 1.06 for mopeds.
The causes of accidents are the conditions of roads, too often inadequate and dangerous for the excessive presence of holes, manholes installed incorrectly and uneven ground. The greatest danger to the cyclist is determined by cars and trucks, which are classified as the most dangerous means. The cause of possible accidents, however, is carelessness of the cyclist or by a dissolute conduct in the management of the two wheels.
Reference is done to previous work for the study of anthropomorphic model of the human figure of a teenager [1] or adult [2], [3], understood as a complex of bones, muscles and joints; for the design of the frame and the bike geometry [5] [11], and finally for the model of the machine [6]. The damage on teenage cyclist is studied because the literature is lacking, while some paper is found for teenagers on scooters [4] or with helmet [32].
The papers on the crash vehicle-cyclist are frequent in the literature, such as [13], [14], [16], [17], [20], many Authors dwell on the comparison of the results on the impact vehicle-cyclist and the vehicle-pedestrian as [15], [19], [21] finally, others Authors [18] dwell in head injury risk under tangential condition or with the analysis of the helmet [31] [32]. Many works are carried out by a statistical analysis of actual accidents, by running programs with evidence of numerical simulation; the most widely used programs are MADYMO and Pc Crash. In [29] Authors conclude that the windscreen is a frequent head and torso impact location; in [30] Authors indicate that car-mounted countermeasures designed to mitigate pedestrian injury have the potential to be effective even for bicyclists. In this paper a contribution is given to an engineering solution for the optimization of cars and bicycles, to limit the damage done by the parties. Simulations are performed using SimWise 4D by DST, alias VisualNastran 4D by MSC.Software, to quantify the damage on the head and chest. Head injuries are studied using HIC criterion while the chest damages are estimated using 3 ms criterion.
2. Indirect approach to vehicle-cyclist crash and damage criteria
The indirect approach has the objective of the reproduction of a cyclist-vehicle accident under certain conditions, faithfully reconstructing the event.
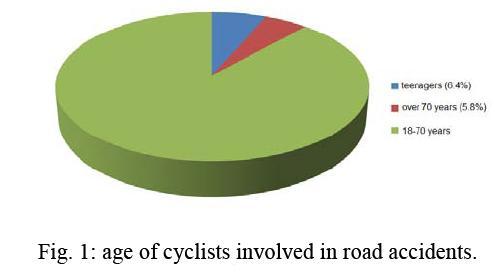
Statistic [9] [10] shows that cyclists, interacting with others and especially with motor vehicles, are just users that least of all respect the rules of the code by adopting often unpredictable behavior. Statistic results are shown in fig. 1-6 and are carried out by analyzing the data of 154 accidents involving drivers of bicycle.
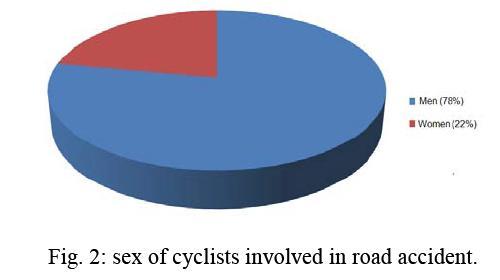
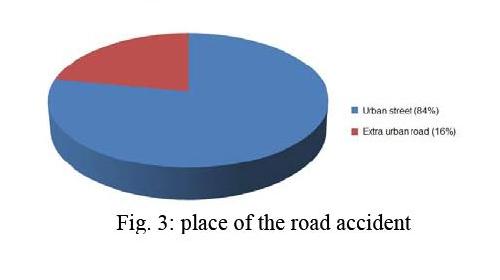
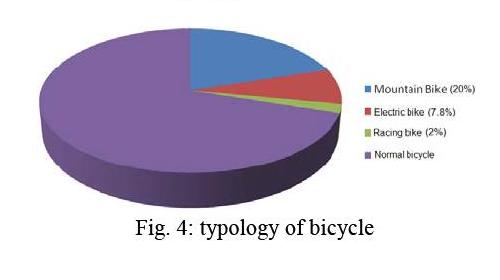
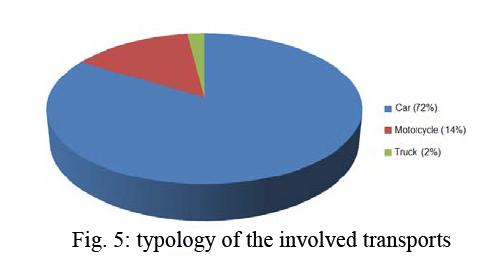
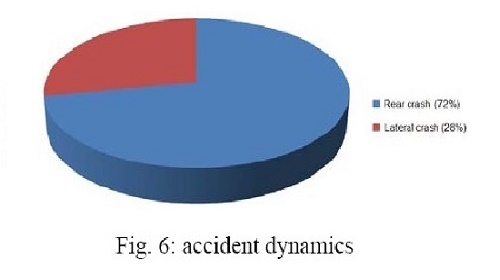
The data, on the last two years, were derived from the archives of the Municipal Police of the City of Palermo and from the archives of three insurance companies. Besides the personal data (age and sex) of the driver, the data are recorded at the accident site (urban, suburban), the type of velocipede, and damage to the vehicles involved, as well as physical damage reported by the cyclist. The most important data concern the injuries sustained by the cyclist; the data are disaggregated taking into account only the most important cause, because the injuries involve multiple body regions. In all cases excoriating lesions and/or contusions are present in various parts of the body (usually upper and lower limbs), they are reported in Fig. 7.
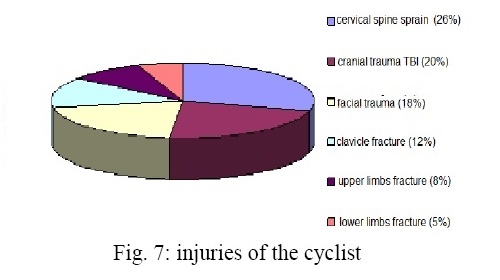
The most relevant data emerge from the integration of the injuries sustained by the cyclist with the accident; moreover the size and location of the lesions can give conclusive information on the speed of impact and on the dynamics (especially in situations with conflicting statements).
Analyzing the available data, the fracture (also of both bones -tibia and fibula) of the legs is achieved by shock with the bumper of the vehicle, or as a result of the fall with flattening of the limb on the ground (in this case the contralateral limb at the point of impact).
The more frequent case is the side impact at low/moderate speed, without loading or projection of the cyclist. Similarly, the isolated fracture of the contralateral clavicle to the impact point is obtained in the fall phase, in crash at low/moderate speeds.
Head injury with or without (dental, maxillo-facial, or nasal bones) fractures, is produced in the phase of loading or projection of the subject. Moreover, injury of the facial mass or multiple injury to the upper limbs (outstretched onward in defense of the noblest districts -head and face) are obtained in cases of telescoping (especially at medium speed). At last, the complex and combined injuries (multiple fractures to the upper and lower limbs, as well as craniofacial trauma) are present in cases of projection, as a result of a telescoping.
3. Injury scale
Most widespread scale of anatomical lesion is AIS, Abbreviated Injury Scale. It classifies the lesions present in a given region of the body through a system of global score based on anatomical aspects. It finds application in forensic medicine to quantify the extent of trauma found on a body so that higher values correspond to more serious injuries AIS. The scale of gravity is ordered in 9 points; the highest score corresponds to a fatal injury. The evaluation of the score of the injury severity is done by dividing the body into six regions, as table 1 shows.
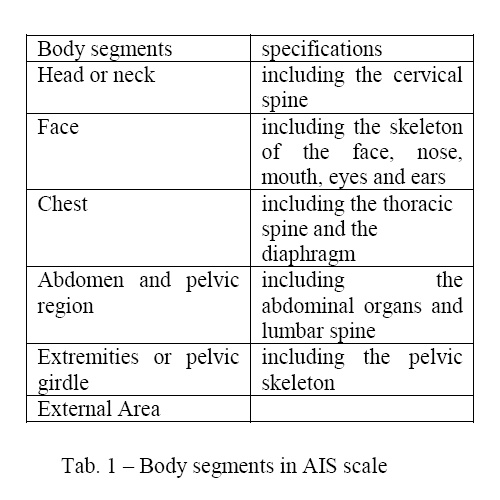
The numerical value of the AIS scale is determined through studies of accident victims whose injuries had already been classified according to the AIS scale. A more detailed specification is found in the international literature, [11] or [1-3]
The risk criteria formulated for the various parts of the body are numerous, the most common are: the Head Injury Criterion (HIC) and Gadd Severity Response (GSR) for the head, Viscous Injury Response (VC ), and 3ms Criterion (3ms) for the chest, the neck, the femurs and tibias, Thoracic Trauma Index (TTI) for the chest. Even in this case the international literature provides more insights on the various risk criteria [1-3] [25] [26]. HIC is used to characterize the injury of the head in the impact of the different zones of the vehicle; it is also used to find correlations between the amount of deformation observed in the vehicle and the magnitude of the acceleration.
According to the directive FMVSS [27], HIC has not to be greater or equal to 1000 over a range of maximum width of 36 ms. It is based on processing the resulting acceleration of the center of gravity of the dummy head, according to the following formula:
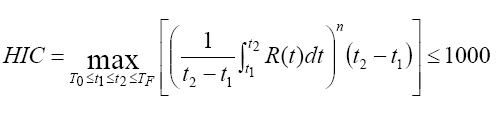
where:
R(t) is the resulting acceleration, in g, measured in the gravity center of the head;
T0 is the time of simulation beginning in seconds;
E il the time of simulation end in seconds;
t1 and t2 represent respectively the initial and final instant of a time interval in seconds; the amplitude of this interval is conventionally equal to 36 ms and it is chosen so that HIC assumes the maximum value.
The acceleration curve is constructed with the experimental values by the accelerometers, and then a sliding time window is applied. The values of HIC are highest in correspondence of the windscreen pillars, of the sides of the hood and the windshield-hood junction area. The range of duration of time is important; the proposal is done to short the interval from 36 to 15 ms [28] in the cases of impact of the head with rigid bodies. HIC equal to 1000 identifies an accident of strong gravity, a value of HIC equal to 2000 has values of gravity more than a thousand, but the severity and probability of lethality of the event are not doubled. Head injuries of AIS scale are classified in tab. 2. The correlation HIC-AIS is only used on the impact test that covers the head; the experiments for the development of this correlation were performed on dead bodies. It is reported for example in the papers [2] and [3].
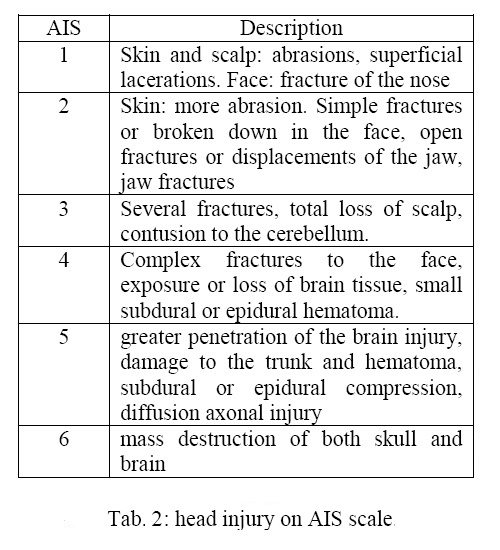
Trauma to the chest may involve the bony wall of the chest, ribs and spine, the pleura, the lung, the diaphragm or the contents of the mediastinum. Due to the potential of anatomical and functional lesion of the coasts and soft tissues, the thoracic injuries are medical emergencies: if not treated quickly and properly can lead to death. The thoracic injuries are from 20% to 25% of all deaths for injury, and complications of thoracic trauma plus 25% of all deaths.
The chest is the only part of the body that also benefits from the lack of seat belt and the air bag; in fact, supported by a seat belt, it suffered a crushing up to 20 mm, with the only belt, 15 mm with the airbag. American standard [7] states that the critical value of crushing of the chest, for the Hybrid III is 76 mm (60g), and then this parameter is not too severe against the driver. In the case of the free dummy the value of crushing is just 3 mm. The most frequent injuries are to the skeleton and soft tissues. Tab. 3 gives a complete overview of the types of injury and the parts involved according to the AIS.
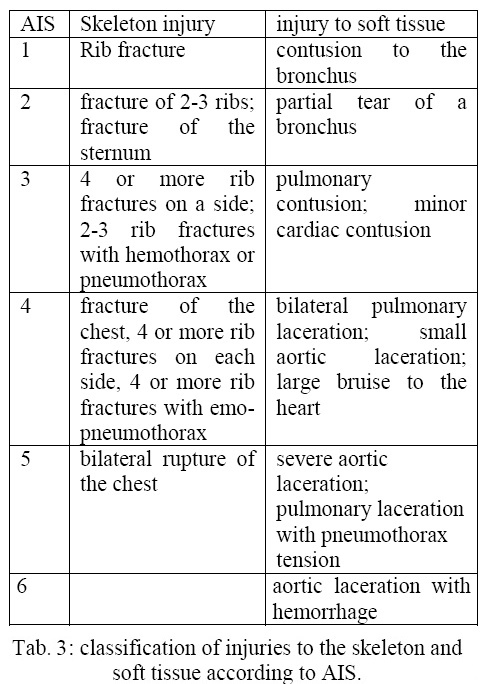
In this paper only 3 ms criterion is used for the chest, because it is particularly useful for the feedback on the cyclist and is used by rules of both the Europe and United States. It prescribes that the centers of gravity of the thorax and the head are not subjected to accelerations above 60 g and 80g, respectively, for a time greater than 3ms. The probability of injury AIS4 + is given by the following expression:

It is equal to 36% assuming gt =60 g.
4. Virtual model
Proser Pro is the ideal animation program for the implementation of the human model; it is effective in this kind of work, especially for the possibility of proportioning of each body segment. The human model represents a teenager, so that the model has a height of 1.45 m and a total mass of 45 kg [1] [6]. The model is imported in Rhinoceros to place a reference system on the center of gravity of the head; each body segment is imported in SimWise4D.
The position of the gravity center of each body segment is entered manually and one by one. To do it, studies conducted on cadavers [22] [23] [24] are taken as a reference.
The car chosen for the simulations is Audi subcompact car. The information on height, length is provided by the manufacturer. This vehicle is chosen for its characteristics: front angle is not much acute and the bonnet is not too high, since the first part of the machine that undergoes in contact is the front bumper in a frontal impact.
The bicycle model is implemented for the study of stability and maneuverability, performing dynamic tests for different geometrical configurations. Element of fundamental importance is its center of gravity, which position significantly influences the dynamic behavior of the bike, especially in accelerating and braking.
To curb the production of bicycles that are too different from each other and to bring the performance differences to the physical abilities of the athletes, the International Cycling Union has placed a limit to the inclination of the upper tube in the design of the frame. Top tube has to fit into a parallelogram having a maximum height of eight centimeters.
5. Simulation of vehicle-cyclist crash
In the general case the teenage cyclist is placed in position perpendicular to the longitudinal axis of the road, and proceeds at a negligible rate in the direction perpendicular to the oncoming vehicle. The action of the vehicle driver takes a decisive role in the evolution of the accident. A speed reduction can only cause minor lesions on the cyclist with respect to a constant speed or higher. In reality the actual decrease in the speed of the car is often very poor: even though the car has a brake power capable of imposing an average deceleration of 0.6g, the effectiveness of braking action would be achieved most of the time near the time of impact.
This paper considers the teenage cyclist in three locations: in the first he is positioned as described above, and then he is on the road with the side facing the vehicle (side impact). In the second case, the cyclist is in front of the vehicle (frontal impact), while in the third and last case the rider is placed behind the vehicle (rear impact or telescoping) Since the law sets the maximum speed equal to 50 km/h on urban road, although the evidence of crash tests complies with this limit. Whereas a speed of 50 km/h can be fatal in the event of impact, crash simulations at speed of 20 km/h, 30 km/h and 40 km/h are also performed.
Parameters measured during the simulations are the accelerations of the center of gravity of both the head and the chest. Fig. 8, Fig. 9 and Fig. 10 show some trends of the acceleration of the head and of the chest versus the time.
Side crashes see a series of peaks of acceleration caused by the impact on the lateral plane of the skull against the front of the vehicle (bonnet and the windscreen), in these cases the first contact with the hood is at the shoulder and in a second time with his head. These peaks are repeated usually in the short round of 0.01s due to some rapid rotation of the head around the joint of the cervical articulation of the neck.
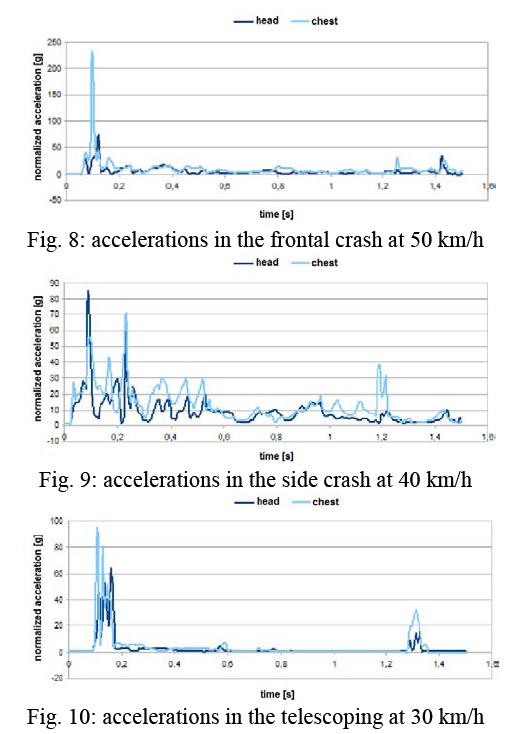
Graphics acceleration -time of front and rear crash depends on the speed assumed for the test so that they are very different at different speeds. It occurs because the head is strongly projected backwards, due to the first contact with the bumper of the vehicle. In this way the center of instantaneous rotation of the cervical articulation varies, by determining a variation of the moment of momentum which results in a substantial increase of the angular acceleration of the head. When there is an overlap of impact of the head and the contact of the chest on the hood, there is a considerable increase of the measured accelerations of the chest. The reconstruction of the events in SimWise under certain conditions and circumstances makes possible to observe the trajectories taken by the adolescent cyclist during the crash.
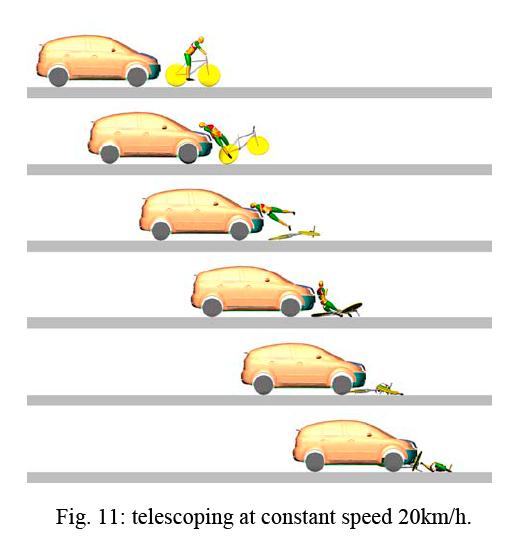
Fig. 11 shows the dynamic rear impact test at 20 km/h; one can note the projection forward of the cyclist.
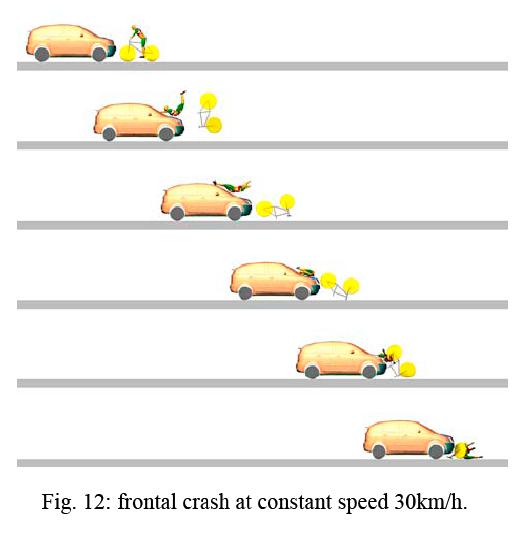
Fig. 12 shows the trajectory of the cyclist in the frontal crash with a vehicle at a constant speed of 30 km/h. One can note the loading on the hood and the gradual release of the body of the rider to the ground.
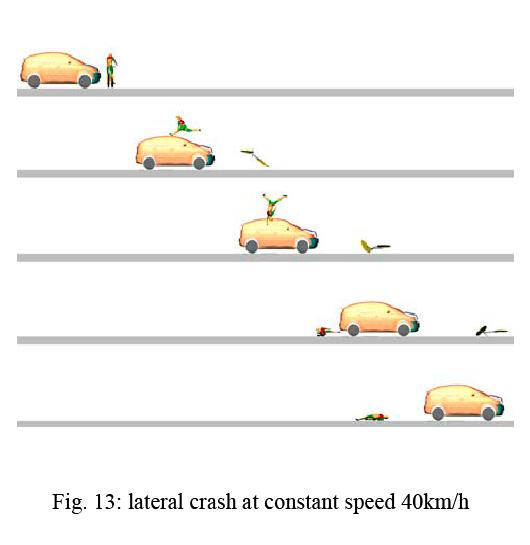
Fig. 13 shows the cyclist in a lateral position regard to the vehicle that proceeds at a constant speed of 40 km/h. One can note the loading phase on the hood and the vaulting of the cyclist.
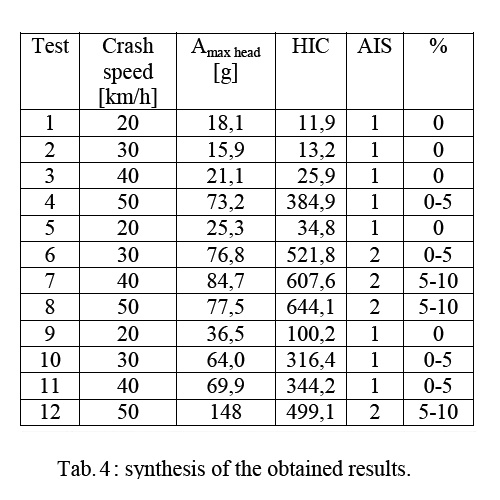
Tab. 4 shows a synthesis of the obtained results and of HIC values.
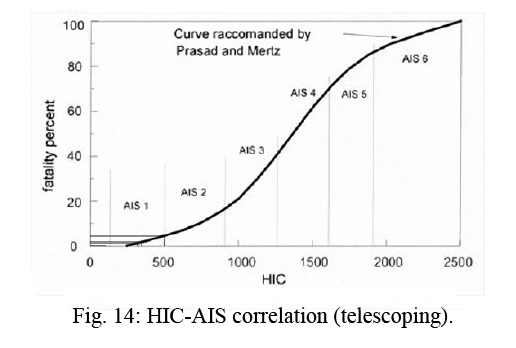
Fig. 14 shows the correlation HIC-AIS in the case of telescoping. HIC data obtained in the simulations together with the scale of lesion AIS determine the percentage of the event fatality. In analogous way the correlation is determined in the other two cases of side and frontal crash. The last two columns of tab. 4 summarize the obtained AIS and lethality percentage.
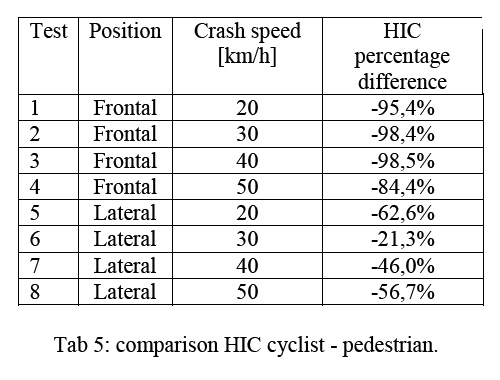
Tab. 5 shows the percentage difference between the impact analysis teenage pedestrian–vehicle and teenage cyclist – vehicle, in terms of HIC.
Fig. 15 and Fig. 16 show the comparison.
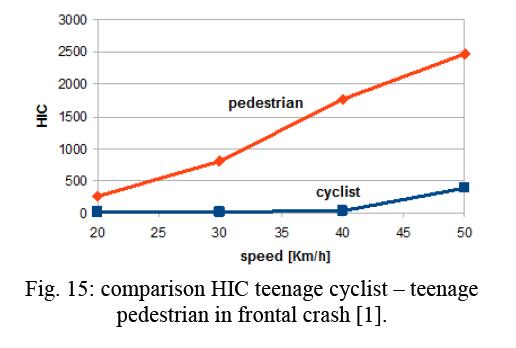
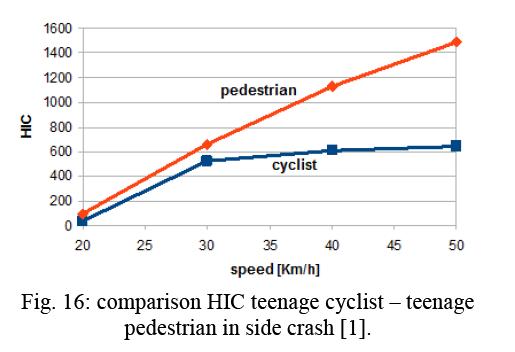
Teenage cyclist has a better chance to survive in frontal and side impact than a pedestrianof the same age because HIC values are consistently lower.
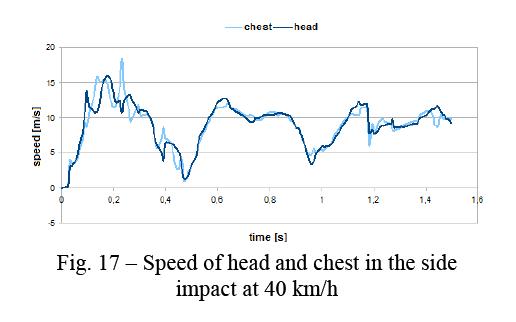
Fig. 17 shows the trend of the speed of both the head and the chest in the case of side impact at 40 km/h versus the time.
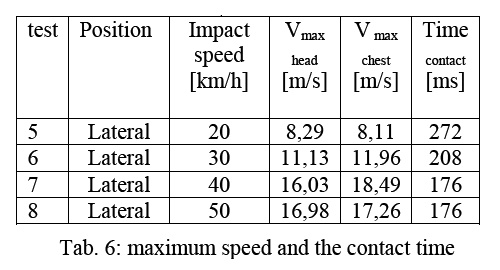
Tab. 6 shows a synthesis of the obtained results and of the values of maximum impact speed with the time of contact.
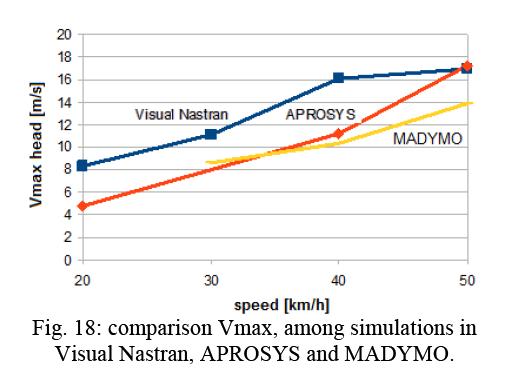
Fig. 18 shows a comparison with analogous data in literature [12], [14] obtained by the software MADYMO, APROSYS and SimWise 4D (alias visualNastran 4D). The data are relative to the case of lateral impact.
The following considerations may be done:
The difference in the value of the maximum speed of impact of the head, regard to the model developed with SimWise 4D, is attributable to the fact that the cyclist examined is a teenager (with mass and height less than that of an adult) and that the front of the vehicles has different geometry.
The impact maximum speed of the head increases as soon as the speed of the vehicle increases.
A virtual accelerometer is added on the gravity center of the chest in order to obtain results of interest for the frontal simulations. Tab. 7 shows the results.
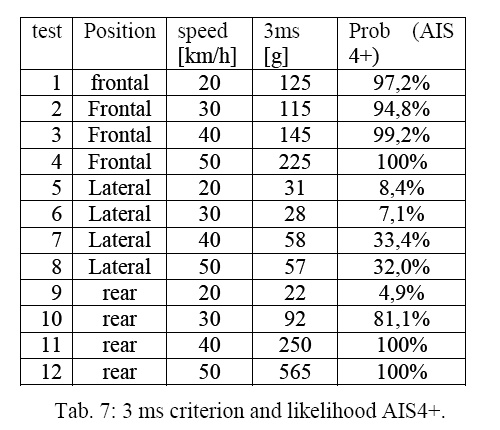
The last column of the table shows the likelihood of injury AIS4 + (fracture of the chest and tearing of the aorta) by making use of the relationship (2). The acceleration values of the chest in the front and back collision are very high. This is due to the capacity of the trunk to flex to direct contact of the chest with the vehicle. Tab. 8 shows the percentage difference between the impact analysis vehicle-teenage cyclist and vehicleteenage pedestrian, following 3 ms criterion.
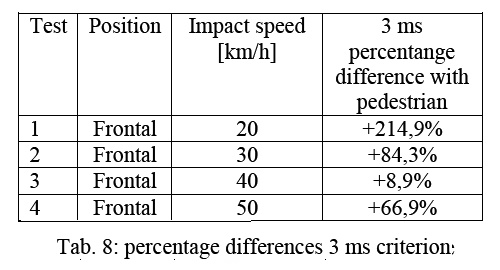
Fig. 19 shows the trend and the comparison.
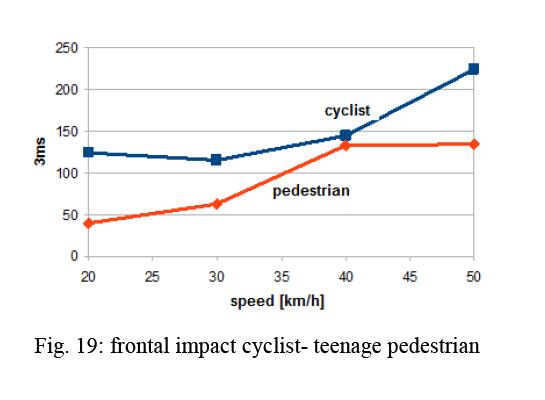
Teenage cyclist is more likely to suffer an injury to the chest, in the frontal impact than a pedestrian of the same age, because the values obtained by 3 ms criterion are consistently greater.
Fig. 20, Fig. 21 and Fig. 22 show the marking of the vehicle for the identification of areas of the bonnet involved when the subject head hits the front of the vehicle (WAD); it occurs according to the directives EURONCAP [8].
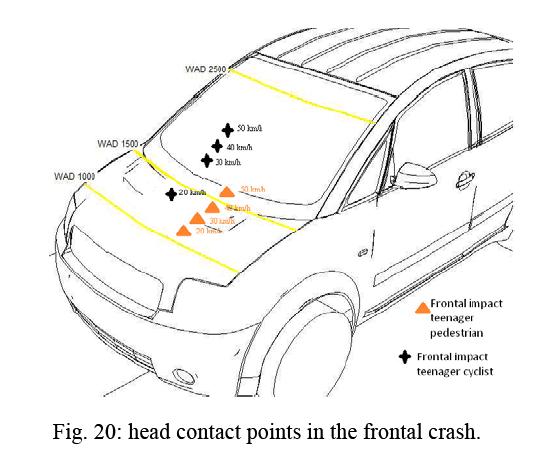
One can observe that the dispersion of the points of impact is localized in all cases in the area of the WAD 1500 except for impacts at 20Km/h and for rear impact vehicle-teenage cyclist at 30km/h (WAD 1000).
Dispersion of points in the side impact involves a larger area than the frontal case. Furthermore, the analysis of the contact points of both cases, allows to obtain a new confirms of the values accuracy. The very intense acceleration peaks correspond to a collision against a rigid wall of the vehicle front.
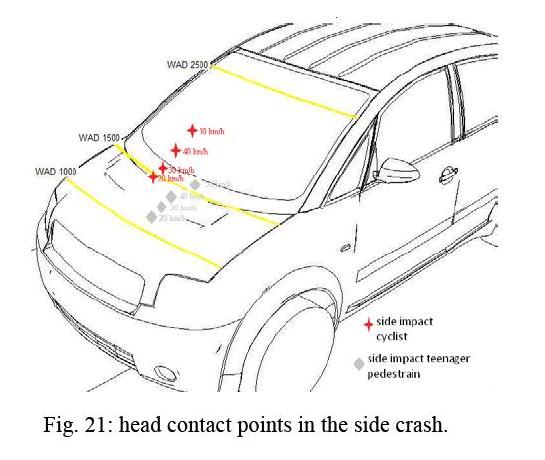
Fig. 20 and fig. 21 compare the points of contact with those of the pedestrian. Simulations stand the same impact zones (WAD 1000), at 20 km/h; the rider hits the underside of the windscreen (areas between 1500-2100 WAD) at 30 and 40 km/h, while the pedestrian hits the upper part of the bonnet (the area between 1000-1500 WAD). Impact points are on the windscreen but at a different height, at 50 km/h. The differences are due to higher position of the head.
6. Conclusions
The aim of this work is the evaluation of the damage in the case of accident in order to suggest improvements and solutions to the designers for the security increase, in order to limit the damage to the person.
The analysis of experimental data and the simulations shows the importance of key elements such as: the height of the rider, the front profile of the car and the minimum height from the ground, the rigidity of the parts that come in contact with the cyclist at the moment of impact. Impact on the bonnet rather than on the windscreen, (it is the case between vehicle and pedestrian), has greater chance to evolve positively, since the bonnet of the car is much less rigid than the windscreen and the percentage of risk of suffering lethal damage is lower. The impact points of the cyclist head are much higher. They occur at a height such as to cause the fall in the vicinity of the windscreen. This difference occurs because the center of gravity of the rider is higher than that of the pedestrian. Position of the rider at the time of the accident is very important: the side position is more damaging than the front, in fact the values obtained from the simulations show that HIC values are higher, because the head of the rider immediately strikes the bonnet; the bike would have to absorb the impact but cannot. A different thing occurs in front and rear impact. In this case the car affects primarily the bike that absorbs the shock, then the impact point is highlighted in the vicinity of the wheel and the cyclist falls in a different way. HIC values are within the value HIC 1000 in all the simulations; this is possible because a good part of the impact is absorbed from the bicycle and not from the body of the cyclist; the contrary occurs in the case of the pedestrian. Higher HIC values are obtained in both front and rear impact of the pedestrian. The values of chest injuries by the criterion of 3ms are above the limit set by rule (60g), they are higher than those resulting from the simulations pedestrian-vehicle. Also the parameter AIS4+ (i.e. fracture of the chest and tearing of the aorta) is higher. Also this time the reason is the center of gravity position of the cyclist; at the moment of impact the chest falls near the windscreen. The use of the multibody software for the simulations is advantageous: in this case the simulations are executed starting from CAD models. In this way, the study of the vehicle, which must necessarily pass approval tests, is certainly easier and can lead to good results with reduction of the costs.
Acknowledgements
Thanks to the Municipal Police of the city of Palermo, the data provided and the friendly collaboration.
References
1. G. Virzì Mariotti, S. Golfo, Determination and analysis of the head and chest parameters by simulation of a vehicle–teenager impact,
Proceedings of the Institution of Mechanical Engineers, Part D: Journal of Automobile Engineering; Vol 228(1), 2014, 3–20
2. Bellavia G., Virzì Mariotti, G., Multibody Numerical Simulation For Vehicle – Pedestrian Crash Test, Ingegneria dell'autoveicolo ATA Vol. 62, 11/12, 2009, pp 40-49 ISSN: 0001-2661; XXI Science and Motor Vehicles 2007, JUMV international Conference with Exhibition, 23-24 April 2007, Belgrade, Serbia, ISBN 978-86-80941-31-8
3. G. Bellavia, G. Virzì Mariotti, Development of an Anthropomorphic model for Vehicle – Pedestrian Crash Test, Ingegneria dell’Autoveicolo, vol. 62, n. 3/4 marzo aprile 2009, pag. 48-56; XXI Science and Motor Vehicles 2007, JUMV international Conference with Exhibition, 23-24 April 2007, Belgrade, Serbia, ISBN 978-86-80941-31-8
4. A. F. Williams, J. Tison, Motor vehicle fatal crash profiles of 13-15-year-olds, Journal of Safety Research, 43, 2012, 145-149
5. F. Giannitrapani, G. Virzi’ Mariotti, Dynamic Analysis of Motorcycle Behaviour on the Road with Steering Plate Structural Optimisation, EAEC Conference, Belgrade, 30th May – 1th June 2005
6. F. P. Giglio, Simulazione numerica dell’impatto veicolo-adolescente con determinazione dei parametri significativi, Undergraduate Thesis, Università di Palermo, Dipartimento di Meccanica, a. a. 2008/09
7. M. Kleinberger, E. Sun, R. Eppinger, S. Kuppa, R. Saul, Development of Improved Injury Criteria for the Assessment of Advanced Automotive Restraint Systems, National Highway Traffic Safety Administration, September 1998
8. EEVC Working Group 17 Report, Improved Test Methods To Evaluate Pedestrian Protection Afforded By Passenger Cars December 1998 with September 2002 updates
9. Istituto Nazionale di Statistica, Rapporto ACIISTAT, Roma, 2012, Italy.
10. L. Ottaviano, D. Palmieri, A. Carnevale, Infortunistica stradale. Aspetti clinico chirurgici, giuridico-assicurativi e medico legali, Giuffré Editore, 2008
11. D. Walder, P. M. Yeoman, A. Turnbull, The abbreviated injury scale as a predictor of outcome of severe head injury, Intensive Care Med 21, 1995: 606-609
12. S. Battaglia, I. Damiani, G. Virzi’ Mariotti, La bicicletta sportiva. Caratteristiche geometriche ed inerziali. Simulazione dinamica, ISBN 88-548-0801-6, Aracne, Roma, 2006.
13. J W Watson, Investigation of Cyclist and Pedestrian Impacts with Motor Vehicles using Experimentation and Simulation, PhD thesis, Cranfield University, feb. 2010
14. Y. Peng, Y. Chen, J. Yang, D. Otte, R. Willinger, A study of pedestrian and bicyclist exposure to head injury in passenger car collisions based on accident data and simulations, Safety Science 50 (9), 2012, 17491759
15. Chen, Q., Chen, Y., Bostrom, O., Ma, Y., Liu, E., A comparison study of car-to-pedestrian and car-to-E-bike accidents: Data source: The China in-depth accident study (CIDAS), SAE Technical Paper 2014-01-0519, 2014, doi:10.4271/2014-01-0519.
16. Xu M. X., Reconstruction analysis of car-electric bicycle side impact accident based on PC-Crash, Journal of Chang'an University (Natural Science Edition), ISSN: 1671-8879, 33, 1, 2013, 85 - 88+99
17. N. Chaurand, P. Delhomme, Cyclists and drivers in road interactions: A comparison of perceived crash risk -Accident Analysis and Prevention 50, 2013, 1176–1184
18. Milne, G., Deck, C., Bourdet, N., (...), Carreira, R. P., Willinger, R., Assessment of bicyclist head injury risk under tangential impact conditions, 2013 IRCOBI Conference Proceedings -International Research Council on the Biomechanics of Injury, pp 735-746.
19. T. Maki, J. Kajzer, K. Mizuno, Y. Sekine, Comparative analysis of vehicle–bicyclist and vehicle–pedestrian accidents in Japan, Accident Analysis & Prevention, Volume 35, Issue 6, 2003, 927–940
20. J.-Ki Kim, S.p Kim, G. F. Ulfarsson, L. A. Porrello, Bicyclist injury severities in bicycle– motor vehicle accidents, Accident Analysis & Prevention 39, 2, 2007, 238–251
21. Y. Peng, Y. Chen, J. Yang, D. Otte, R. Willinger, A study of pedestrian and bicyclist exposure to head injury in passenger car collisions based on accident data and simulations, Safety Science 50, 9, 2012, 1749– 1759
22. Yeadon, M. R., The simulation of aerial movement. I, II, III., IV, Journal of Biomechanics 23 (1), 1990, pp. 56-89
23. Braune, W., Fisher, O., Über den Schwerpunkt des menschlichen Körpers, mit rucksicht auf die Ausrüstung des deutschen Infanteristen, ABH Math Phy Cl K Sachs Ges Wissensch 15, 1889, pp. 559 2
24. Kaleps, I., Clauser, C.E., Young, J.W., Investigation into the mass distribution properties of the human body and its segments, Ergonomics 27. 12, 1984, pp. 12251237 8
25. Schmitt K. U., Niederer P. F., Muser M. H., Walz F., Trauma Biomechanics: Accidental injury in traffic and sports, Springer London, 2007
26. A. M. Nahum, J. W. Melvin, Accidental Injury: Biomechanics and Prevention Springer, London, 2001
27. FMVSS 201U, Occupant Protection in Interior Impact. Upper Interior Head Impact Protection, April 3, 1998
28. U.S. Department of Transportation, NHTSA, http://www.nhtsa.gov/cars/rules/.../PEA/peaIII.n.pdf
29. M. van Schijndel, S. de Hair, C. Rodarius, R. Fredriksson, Cyclist kinematics in car impacts reconstructed in simulations and full scale testing with Polar dummy, IRC-12-85 IRCOBI Conference 2012, pp 800-812
30. R. Fredriksson, E. Rosén, Priorities for Bicyclist Protection in Car Impacts – a Real life Study of Severe Injuries and Car Sources, IRC-12-85 IRCOBI Conference 2012, pp 779786
31. Cripton P. A., Dressler D. M., Stuart C. A., Dennison C. R., Richards D., Bicycle helmets are highly effective at preventing head injury during head impact: head-form accelerations and injury criteria for helmeted and unhelmeted impacts. Accident Analysis and Prevention. 70, 2014, 1-7. doi: 10.1016/j.aap.2014.02.016.
32. Mizuno K, Ito D, Yoshida R, Masuda H, Okada H, Nomura M, Fujii C., Adult headform impact tests of three Japanese child bicycle helmets into a vehicle. Accident Analysis and Prevention 73C, 2014, 359-372, doi: 10.1016/ j.aap.2014.09.018
OTHER 83 DIFFERENT APPLICATIONS !
ALTRI 83 CASI IN SETTORI DIVERSI !